Elements
Virtual Particles and Quarks etc
Are virtual particles really constantly popping in and out of existence?
Gordon Kane, director of the Michigan Center for Theoretical Physics at the University of Michigan at Ann Arbor, provides this answer. Scientific American
Virtual particles are indeed real particles. Quantum theory predicts that every particle spends some time as a combination of other particles in all possible ways. These predictions are very well understood and tested.
Quantum mechanics allows, and indeed requires, temporary violations of conservation of energy, so one particle can become a pair of heavier particles (the so-called virtual particles), which quickly rejoin into the original particle as if they had never been there. If that were all that occurred we would still be confident that it was a real effect because it is an intrinsic part of quantum mechanics, which is extremely well tested, and is a complete and tightly woven theory--if any part of it were wrong the whole structure would collapse.
But while the virtual particles are briefly part of our world they can interact with other particles, and that leads to a number of tests of the quantum-mechanical predictions about virtual particles. The first test was understood in the late 1940s. In a hydrogen atom an electron and a proton are bound together by photons (the quanta of the electromagnetic field). Every photon will spend some time as a virtual electron plus its antiparticle, the virtual positron, since this is allowed by quantum mechanics as described above. The hydrogen atom has two energy levels that coincidentally seem to have the same energy. But when the atom is in one of those levels it interacts differently with the virtual electron and positron than when it is in the other, so their energies are shifted a tiny bit because of those interactions. That shift was measured by Willis Lamb and the Lamb shift was born, for which a Nobel Prize was eventually awarded.
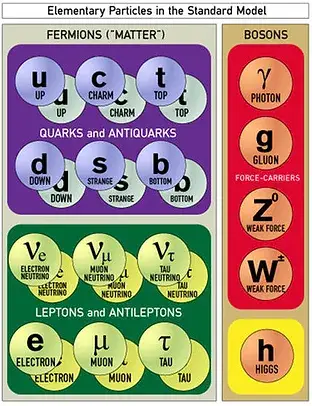
Quarks (Ferrmions)
Quarks are particles much like electrons, but different in that they also interact via the strong force. Two of the lighter quarks, the so-called "up" and "down" quarks, bind together to make up protons and neutrons. The "top" quark is the heaviest of the six types of quarks. In the early 1990s it had been predicted to exist but had not been directly seen in any experiment. At the LEP collider at the European particle physics laboratory CERN, millions of Z bosons--the particles that mediate neutral weak interactions--were produced and their mass was very accurately measured. The Standard Model of particle physics predicts the mass of the Z boson, but the measured value differed a little. This small difference could be explained in terms of the time the Z spent as a virtual top quark if such a top quark had a certain mass.
When the top quark mass was directly measured a few years later at the Tevatron collider at Fermi National Accelerator Laboratory near Chicago the value agreed with that obtained from the virtual particle analysis, providing a dramatic test of our understanding of virtual particles.
Another very good test some readers may want to look up, which we do not have space to describe here, is the Casimir effect, where forces between metal plates in empty space are modified by the presence of virtual particles.
Thus virtual particles are indeed real and have observable effects that physicists have devised ways of measuring. Their properties and consequences are well established and well understood consequences of quantum mechanics.
Leptons
Leptons are elementary particles that exist on their own. Leptons do not take part in the strong interaction, and only interact via the electromagnetic and weak forces.
There is evidence for six different kinds of leptons – three negatively electrically charged leptons, and three electrically neutral. The best known electrically charged leptons are:
-
the electron (e)
-
the muon (μ)
-
the tauon (τ)
The three electrically neutral leptons are the neutrinos (ν). Associated to each charged lepton, there are three distinct kinds of neutrinos:
-
the electron neutrino (νe)
-
the muon neutrino (νμ)
-
the tauon neutrino (νε)
Neutrinos are produced in a variety of interactions. The sun produces millions of neutrinos in the internal fusion reactions that power it.
Since neutrinos do not interact electrically or strongly, they almost never interact with any other particle. Most neutrinos pass right through the Earth without ever interacting with any single atom.
One piece of evidence comes from the measured hydrogen/helium abundance ratio in the universe. When the process of nuclei formation (nucleosynthesis) from the Big Bang is modelled, the number of types of neutrinos affects the abundance of helium. The observed abundance agrees with three types of neutrinos.
Read more at: https://phys.org/news/2014-01-leptons.html#jCp
Gauge Bosons
The Standard Model of elementary particles, with the gauge bosons in the fourth column in red.
In particle physics, a gauge boson is a force carrier, a bosonic particle that carries any of the fundamental interactions of nature, commonly called forces. Elementary particles, whose interactions are described by a gauge theory, interact with each other by the exchange of gauge bosons—usually as virtual particles.
Gauge bosons are different from the other kinds of bosons: first, fundamental scalar bosons (the Higgs boson); second, mesons, which are composite bosons, made of quarks; third, larger composite, non-force-carrying bosons, such as certain atoms. The Standard Model of particle physics recognizes four kinds of gauge bosons: photons, which carry the electromagnetic interaction; W and Z bosons, which carry the weak interaction; and gluons, which carry the strong interaction.
On 4 July 2012, the ATLAS and CMS experiments at CERN's Large Hadron Collider announced they had each observed a new particle in the mass region around 126 GeV. This particle is consistent with the Higgs boson predicted by the Standard Model. The Higgs boson, as proposed within the Standard Model, is the simplest manifestation of the Brout-Englert-Higgs mechanism. Other types of Higgs bosons are predicted by other theories that go beyond the Standard Model. An ATLAS candidate event for the Higgs boson particle decaying into two bottom quarks. Physicists at CERN recently observed this process, which further confirms the Standard Model of particle physics.Aug 29, 2018
Particle Masses
Particles are built by Quarks binding together to form different particles which, in turn, are formed by the action of quantum fluctuations which require energy to bind the Quarks together. The binding energy is held by Flux Tubes which link the Quarks together.
Protons and Neutrons are are made up from Quarks. Quark masses are so small that they only make up about 1% of the mass of the proton (and a similar fraction of the neu_tron). The rest of the mass comes from the energy in the gluon field . Gluons are massless, but there is so much energy in the field that by E=mc^2 there is a significant amount of mass there, i.e. m=E/c^2. This is where most of the proton's mass (hence your mass) comes from and the mass of virtually everything around you. The Higgs mechanism only applies to fundamental particles, which means that electrons derive their mass entirely from the Higgs interaction but protons and neutrons, made of quarks, do not.
See the video below for a graphical description:
https://www.youtube.com/watch?time_continue=148&v=Ztc6QPNUqls&feature=emb_logo
Create Your Own Website With Webador