The Character of the Cosmos
Understanding the Cosmic Microwave Background
The cosmic microwave background (CMB) is the afterglow of the Big Bang, and it can be thought of as a snapshot of the early universe. Scientists have measured the CMB using sensitive instruments like the Planck satellite, which has provided valuable insights into the origins and evolution of the cosmos.
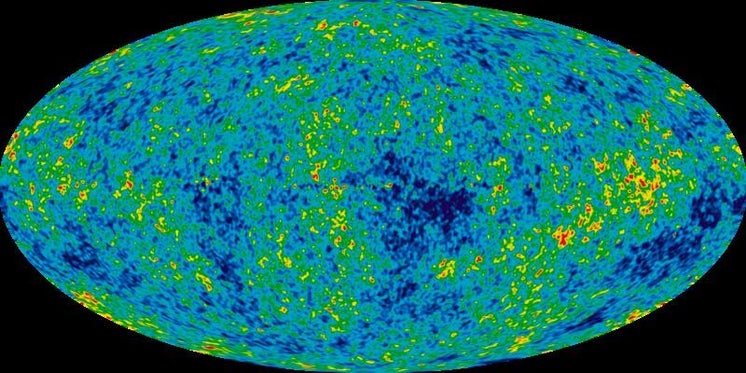
The CMB is landmark evidence of the Big Bang theory for the origin of the universe. In the Big Bang cosmological models, during the earliest periods, the universe was filled with an opaque fog of dense, hot plasma of sub-atomic particles. As the universe expanded, this plasma cooled to the point where protons and electrons combined to form neutral atoms of mostly hydrogen. Unlike the plasma, these atoms could not scatter thermal radiation by Thomson scattering, and so the universe became transparent. Known as the recombination epoch, this decoupling event released photons to travel freely through space. However, the photons have grown less energetic due to the cosmological redshift associated with the expansion of the universe. The surface of last scattering refers to a shell at the right distance in space so photons are now received that were originally emitted at the time of decoupling.
The CMB is not completely smooth and uniform, showing a faint anisotropy that can be mapped by sensitive detectors. Ground and space-based experiments such as COBE, WMAP and Planck have been used to measure these temperature inhomogeneities. The anisotropy structure is determined by various interactions of matter and photons up to the point of decoupling, which results in a characteristic lumpy pattern that varies with angular scale. The distribution of the anisotropy across the sky has frequency components that can be represented by a power spectrum displaying a sequence of peaks and valleys. The peak values of this spectrum hold important information about the physical properties of the early universe: the first peak determines the overall curvature of the universe, while the second and third peak detail the density of normal matter and so-called dark matter, respectively. Extracting fine details from the CMB data can be challenging, since the emission has undergone modification by foreground features such as galaxy clusters.
Multiverse Theory
Multiverse theory suggests that our universe, with all its hundreds of billions of galaxies and almost countless stars, spanning tens of billions of light-years, may not be the only one. Instead, there may be an entirely different universe, distantly separated from ours — and another, and another. Indeed, there may be an infinity of universes, all with their own laws of physics, their own collections of stars and galaxies (if stars and galaxies can exist in those universes), and maybe even their own intelligent civilizations.
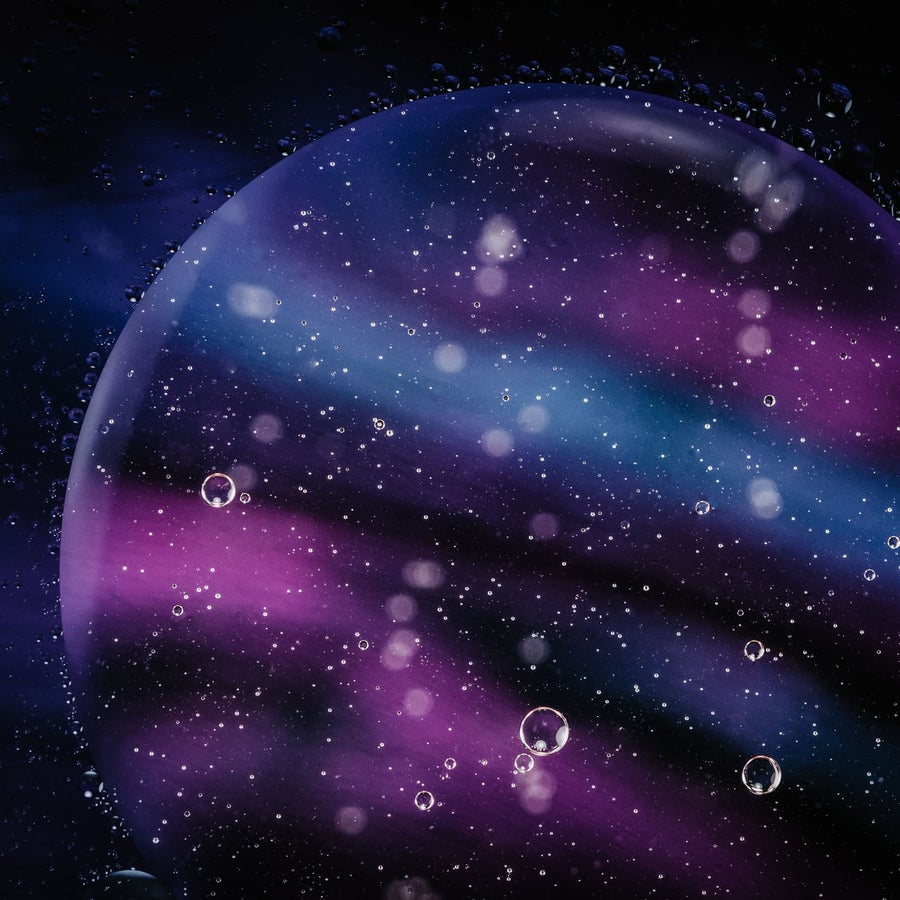
The concept of the multiverse arises in a few areas of physics (and philosophy), but the most prominent example comes from something called inflation theory. Inflation theory describes a hypothetical event that occurred when our universe was very young — less than a second old. In an incredibly brief amount of time, the universe underwent a period of rapid expansion, "inflating" to become many orders of magnitude larger than its previous size.
While inflation ended in our universe, there may have been other, much more distant regions where inflation continued — and continues even today. Individual universes can "pinch off" of larger inflating, expanding universes, creating an infinite sea of eternal inflation, filled with numerous individual universes.
In this eternal inflation scenario, each universe would emerge with its own laws of physics, its own collection of particles, its own arrangement of forces and its own values of fundamental constants. This might explain why our universe has the properties it does — particularly the properties that are hard to explain with fundamental physics, such as dark matter or the cosmological constant, Deng said.
"If there is a multiverse, then we would have random cosmological constants in different universes, and it is simply a coincidence that the one we have in our universe takes the value that we observed," he said.
It’s worth revisiting Hawking’s appearance at the Seattle Science Festival on June 16, 2012, when he gave a talk at the Paramount Theater titled “Brane New World.”
In that talk, visible in the Pacific Science Center’s video above and transcribed below, Hawking laid out his views on what he thought could be the ultimate theory of the universe, known as M-theory.
GeekWire’s Alan Boyle covered the event for NBCNews.com and wrote at the time that Hawking’s “crazy smarts and his sharp wit” were both in evidence that night.
Here is the transcription:
Brane New World
“In this talk I want to describe an exciting development that may transform our view of the universe and of reality itself, whatever that may be. The idea is that we may live on a brane, or surface, in a larger space. The word ‘brane,’ spelled b-r-a-n-e, was introduced by my colleague Paul Townsend to indicate decentralization of the membrane to more dimensions.
“I suspect the pun on ‘brain’ was quite deliberate.
“We think we live in three-dimensional space that is weak and specifies the position of an object in the room by three numbers. There could be five feet from the north wall, three feet from the east wall, and two feet above floor level.
“Or on a large scale, there could be latitude, longitude and height above sea level. On an even larger scale, we can specify the position of a star in the galaxy by three numbers: galactic latitude and longitude, and distance from the center of the galaxy.
“As well as the three numbers that specify position, we can add a fourth number that specifies time. Thus we can describe ourselves as living in a four-dimensional spacetime, where events are labeled by four numbers: three for the the position of the event and the fourth for its time.
“It was Einstein’s stroke of genius to realize that spacetime isn’t flat but is curved and warped by the matter and energy in it. According to this general theory of relativity, objects like planets try to move on straight lines through spacetime, but because spacetime is curved, their paths appear bent as if by a gravitational field. It is as if you placed a heavy weight to represent a star on a sheet of rubber. The weight will depress a sheet and cause it to curve near the star.
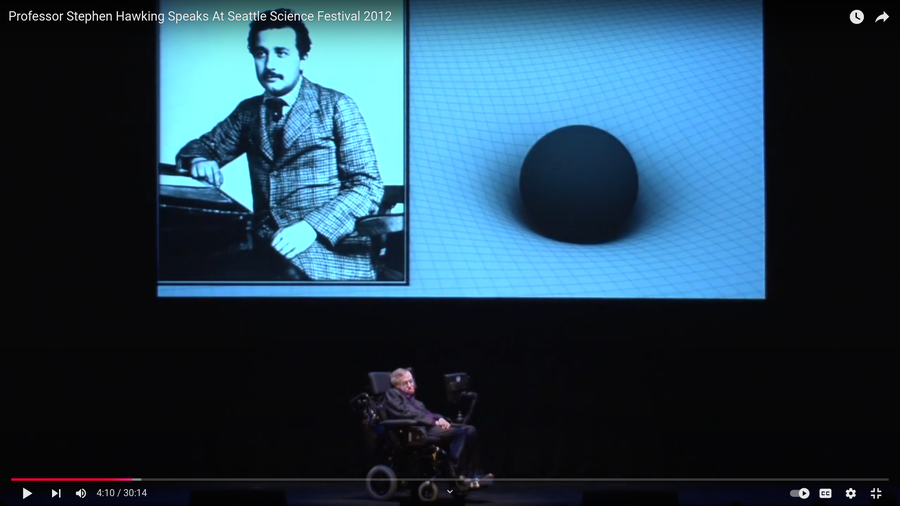
“If you now roll little ball bearings on the sheet to represent planets, they can orbit a star.
“We now have confirmation that spacetime is curved, from the GPS navigation system which is fitted to boats, airplanes and some cars. This operates by comparing signals from several satellites. If one assumed spacetime was flat, one would calculate the wrong position.
“Three(Pacific Science Center / YouTube screen grab) dimensions of space and one of time are all that we see. So why should we believe in extra dimensions that we can observe? Are they just science fiction, or do they have detectable consequences?
“The reason we take the idea of extra dimensions seriously is that, although Einstein’s general theory of relativity agrees with all of the observations we have made, the theory predicts its own downfall. Roger Penrose and I showed that it predicted that time had a beginning and a big bang, and an end in black holes. At these places, the general theory of relativity would break down, so one could not use it to predict how the universe began, or what would happen to someone who fell in a black hole.
Many scientists have tried to find more physical, hard evidence for the multiverse's existence. For example, if a neighboring universe happened to be close to ours long ago, it may have collided with our universe, creating a detectable imprint. That imprint could be in the form of distortions in the cosmic microwave background (the light left over from when the universe was a million times smaller than it is today) or in strange galaxy properties in the direction of the collision, according to the Early Universe blog published by University College London but all of these types of searches have come up empty, so the multiverse remains hypothetical.
Related: If we live in a multiverse, where are these worlds hiding?
Deng is searching for evidence of the multiverse by looking for special kinds of black holes that could be artifacts of pieces of our universe that separated into their own universe via a process called quantum tunneling. If some regions of our universe separated this way, they would have left behind "bubbles" in our universe that would turn into these unique black holes, which may still exist today, according to Deng.
"The potential detection of these black holes can then point to the existence of a multiverse," Deng said.
Revealing the Cosmic Web
The cosmic web is a vast network of filaments and clusters that connect galaxies across the universe. Modern telescopes like the Hubble Space Telescope have allowed us to visualize this intricate structure, shedding light on the distribution of matter and energy in the cosmos.
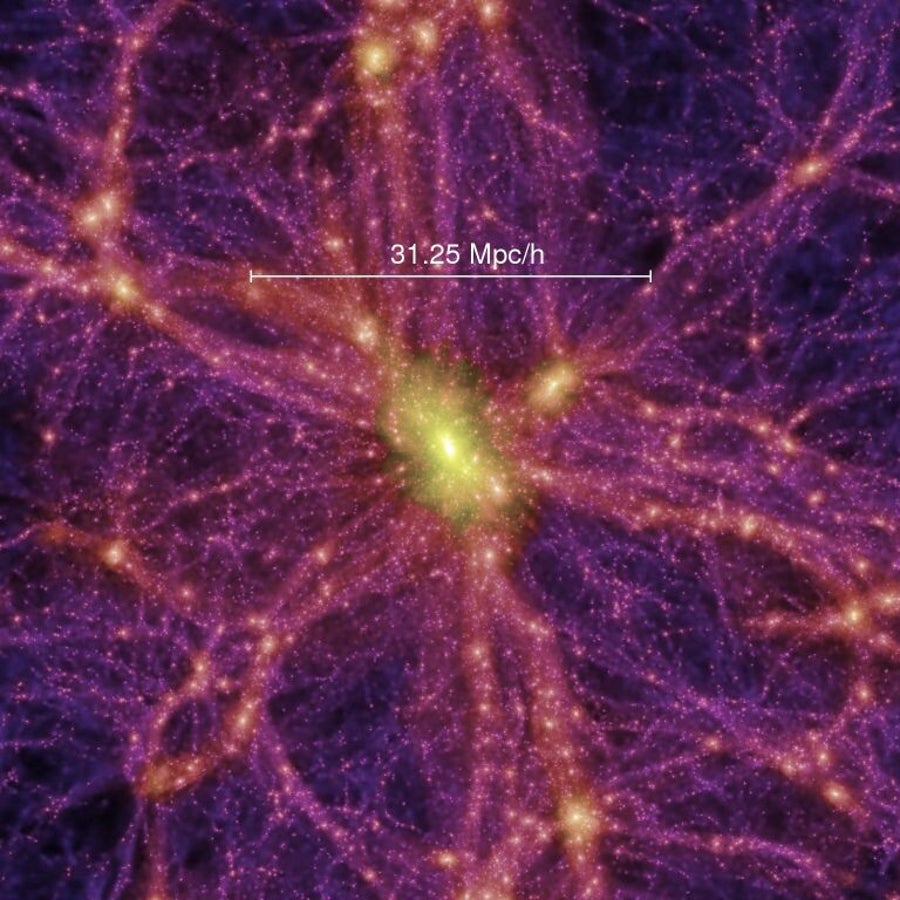
The Cosmic Microwave Background (CMB) is the cooled remnant of the first light that could ever travel freely throughout the Universe. This 'fossil' radiation, the furthest that any telescope can see, was released soon after the Big Bang. ESA's Planck mission detected this first light, which is also the 'oldest' radiation detectable and carries information about our past and future. By observing it, Planck saw the Universe as it was almost at its origin.
The CMB radiation was discovered by chance in 1965. Penzias and Wilson, two radio astronomers in the United States, registered a signal in their radio telescope that could not be attributed to any precise source in the sky.
It apparently came from everywhere with the same intensity, day or night, summer or winter. They concluded that the signal had to come from outside our Galaxy. It came almost from the origin of the Universe.
Scientists have recently identified a connection between metal-poor regions in a set of dwarf galaxies and bursts of star-formation activity within them. These observations provide long-awaited evidence supporting predictions of how stars formed in the early universe and in dwarf galaxies today.
Metal-Poor Clues
The primary driver of star formation over cosmic history is thought to be the accretion onto galaxies of cold gas streaming from the cosmic web. The best way to confirm this model would be to observe a cloud of cosmic gas flowing into an otherwise-quiescent galaxy and launching a wave of star formation. But because cold gas doesn’t emit much radiation, it’s difficult to detect directly.
Now, a team of scientists have found a clever way around this problem: they searched galaxies for a correlation between areas of active star formation and metal-poor regions. Why? Because metal-poor regions could be a smoking gun indicating a recently accreted cloud of cold gas from the cosmic web.
The Expanding Universe
Since the Big Bang, the universe has been steadily expanding, carrying galaxies further apart from each other. Not only that but they have been accelerating faster and faster away from us. The timeline of this expansion, marked by key events like the formation of stars and galaxies, shapes the character of the cosmos we observe today.
We must not forget that our view of the universe is in the past and furthest objects have already been created billions of years ago. That means that we are part of the new creation and this is where expansion is taking place! now. It is a relative viewpoint that we have.
Unravel the Mysteries of the Universe
Delve into the wonders of the cosmos. Join us on a journey of discovery and exploration.
Create Your Own Website With Webador